Research Home
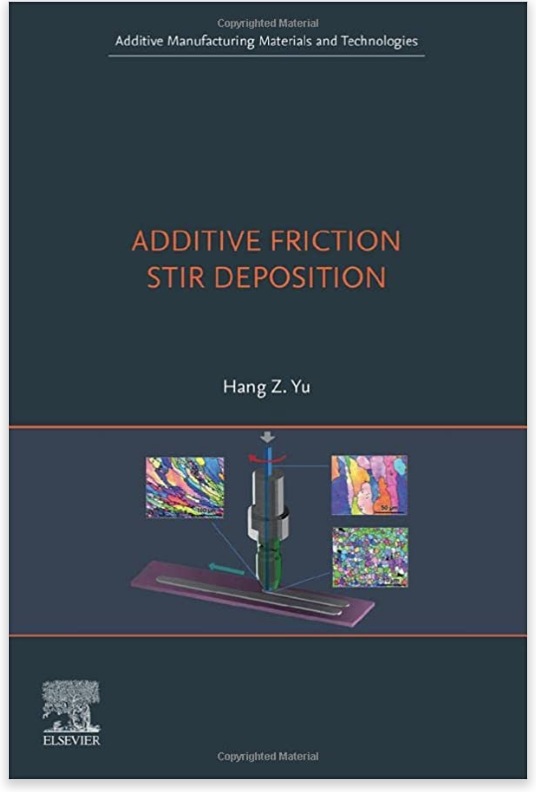
The Yu Research Group conducts research in the realm of Materials Processing Science and Additive Manufacturing:
1. Solid-state metal additive manufacturing enabled by additive friction stir deposition. This process has demonstrated salient advantages over mainstream fusion-based metal additive manufacturing in quality, properties, cost, energy consumption, and environmental impact. Characterized by rapid and extensive shear flow at elevated temperatures along with continual and sufficient energy supply, the system operates under a far-from-equilibrium, 'driven' condition, which cannot be described using linear irreversible thermodynamics applicable to near-equilibrium scenarios.
Through in situ or time-resolved characterization and theoretical innovation, we aim to develop an in-depth understanding of the unique kinetic phenomena underlying such driven conditions, ranging from atomic transport to phase/microstructure evolution. Examples of interest include continuous dynamic recrystallization, shear-induced mixing and segregation at the atomic scale, and shear-induced fracture and dispersion at the micro- and meso-scale. With the gained process and materials science fundamentals, we seek to establish quantitative process-structure-property linkages and advance material sustainability through niche applications, such as solid-state rapid metal recycling, structural repair, and resilient manufacturing (in austere conditions). Additionally, through collaboration, we are exploring quality control enabled by the integration of in situ monitoring, physics simulation, and AI-based prediction.
Our research portfolio covers process fundamentals (temperature, material flow), dynamic phase and microstructure evolution mechanisms, design and manufacturing of heterostructured materials, and circular economy applications such as structural repair and recycling (and upcycling). Materials of interest include precipitate-strengthened alloys (e.g., aerospace grade 7xxx and 2xxx Aluminum), two-phase alloys (e.g., Ti-6Al-4V), pure metals (e.g., Cu), as well as functional materials, such as magnetic materials and shape memory alloys and composites. We have done pioneering work on this topic as elaborated below.
* Process fundamentals. The Yu research group has established an in situ monitoring platform for additive friction stir deposition, which provides real-time information on temperature field of the printed material, materials flow, applied force and torque, and substrate temperature. Based on that, the relationship between the thermal characteristics (e.g., peak temperature, exposure time, heating/cooling rate) and processing variables has been quantitatively established for the first time (Additive Manufacturing 34, 101386, 2020; lead author: Ph.D. student David Garcia). Using tracer-based X-ray computed tomography in dissimilar metal printing, we have discovered unique 3D features forming at the interface with significant macroscopic material mixing. From these observations, the mechanisms for interfacial material flow and interface morphology formation are elucidated (Additive Manufacturing 35, 101293, 2020; lead author: Ph.D. student Mackenzie Perry). By engineering the feed materialand tracking the tracer shape evolution using X-ray computed tomography, the strain development process during additive friction stir deposition is quantified, wherein the total strain is estimated to be on the order of 10 or higher (Materialia, Volume 18, August 2021, 101159; lead author: Ph.D. student Mackenzie Perry, who won the Acta student award based on this paper) . We have also recently developed a process model based on the Non-Newtonian fluid assumption, from which we are able to unravel the material flow path of individual voxels (Journal of Manufacturing Processes, Volume 101, 114-127, 2023). By integrating the in situ monitoring, time-resolved characterization results with process modeling, we have collaborated with Prof. David Higdon to develop a physics-informed data-driven model to efficiently predict the entire thermal (T vs. t) and deformation (ε̇ vs. t) history as a function of processing variables (Journal of Manufacturing Systems, Volume 72, 1-15, 2024).
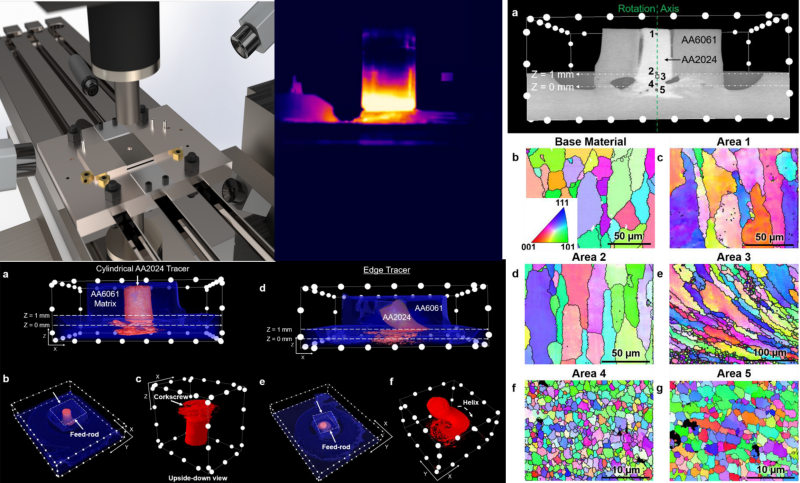
*Microstructure and properties. We have compared the process-microstructure linkages and dynamic microstructure evolution mechanisms between materials of high and medium stacking fault energy. The intrinsic thermomechanical properties and strain development through tool-material interactions have been identified as the key factors for final microstructures in additive friction stir deposition (Materialia, 15, 100967, 2020; lead author: Ph.D. student Joey Griffiths). We have developed an economical solution for printing high-strength aluminum using additive friction stir deposition. The printed AA7075 is shown to exhibit wrought-like mechanical properties, with yield strength of 477 MPa, ultimate tensile strength of 541 MPa, and elongation of 8.2%. Without adding nanoparticles, such values are notably higher than those published for fusion-based additive manufacturing (Materials & Design, 198, 109288, 2020; lead author: Ph.D. student Jake Yoder). We have demonstrated forging standard properties in the as-printed Ti-6Al-4V both along the in-plane and out-of-plane directions via closed-loop temperature control (Journal of Materials Processing Technology, Volume 322, 118201; lead author: Ph.D. student Jake Yoder). At this moment, we are working on a series of publications on dynamic phase and microstructure evolution during additive friction stir deposition, covering kinetic phenomena such as shear-induced mixing, shear-induced segregation, shear-induced second-phase particle fracture and dispersion, heterogeneous nucleation, and loss of interface coherency.
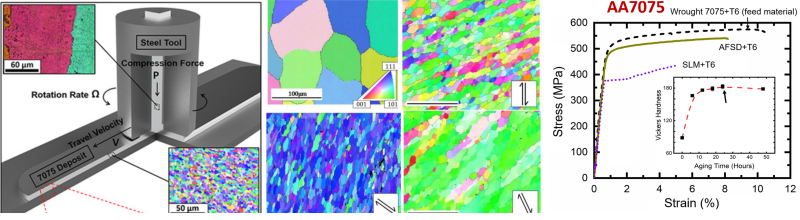
* Niche engineering applications. We have employed additive friction stir deposition for additive repair of structural components, showing that deep holes and grooves are readily filled with good interface bonding and mixing (Applied Sciences, 9, 3486, 2019; lead author: Ph.D. student Joey Griffiths). We have also published a viewpoint paper discussing the promise of using solid-state metal additive manufacturing, espeically cold spray and additive friction stir deposition, for structural repair (Acc. Mater. Res. 2021, 2, 9, 780–792; lead author: M. S. student Ryan Gottwald) . Together with industrial collaborators from Ford Motor Company, our research group has developed a method for selective-area cladding on thin automotive sheet metals without local buckling. This work also allows for a first assessment of residual stress levels for this emerging process (Journal of Materials Processing Technology, 291, 117045, 2021; lead author: Ph.D. student Doug Hartley). Our group has recently demonstrated underwater additive manufacturing using additive fricton stir deposition, paving the road towards metal additive manufacturing under austere conditions (Materials and Design, 111148, 2022; lead author: Ph.D. student Joey Griffiths). Through collaboration with Nissan, we show the upcycling effect in additive friction stir deposition of cast Al chips, highlighting the potential for secondary feedstock usage admist of supply chain disruptions (Additive Manufacturing Letters, 100108, 2023; lead author: Ph.D. student Jake Yoder).
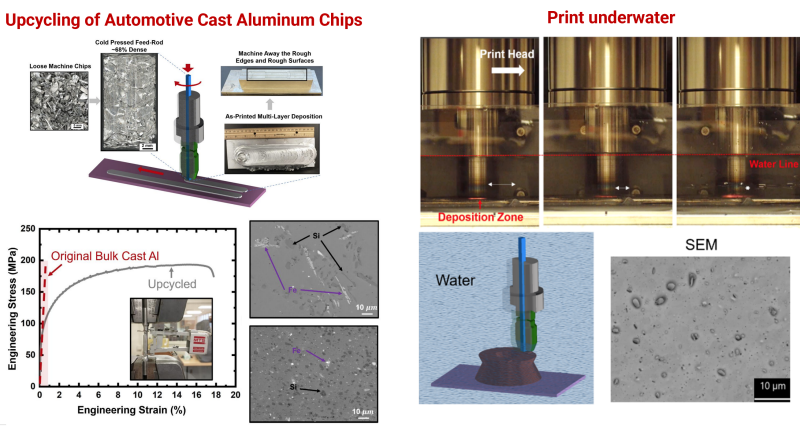
2. Shape memory ceramics. Through the pioneering use of in situ neutron diffraction to study zirconia-based shape memory ceramics, we have discovered a series of intriguing behaviors in the granular form of these materials, especially the ‘continuous’ mode of transformation that occurs over a wide range of stress and temperature. This has subverted the traditional understanding of martensitic transformation, offering new strategies for controlling the transformation mode through mesostructure and mechanical constraint. Relevant publications: Journal of Applied Physics, 128, 245102, 2020 (Editor’s Pick; lead author: Ph.D. student Hunter Rauch), Acta Materialia, 168, 362-375, 2019 (lead author: Ph.D. student Hunter Rauch), and Acta Materialia, 132, 455-466, 2017. In collaboration with Prof. Rayne Zheng at UC Berkeley, our group recently developed a process for 3D printing zirconia and examined the mechanical and transformation behavior in the printed Y-TZP micro-honeycombs (Additive Manufacturing, 102692 (2022); lead author: Ph.D. student Hunter Rauch).
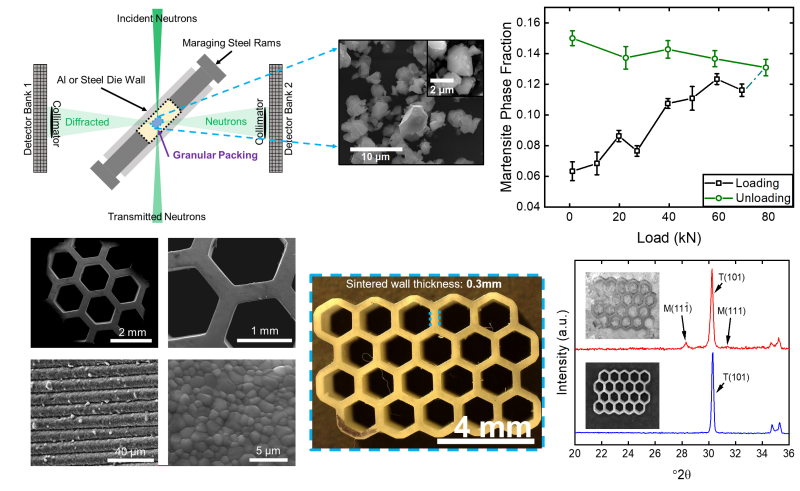
3. Materials design for additive manufacturing. By integrating physically-based and data-driven modeling with experiments, mesostructure optimization has been implemented and validated for multi-material additive manufacturing, and thermal field evolution has been accurately predicted in additive manufacturing. Relevant publications: Materials & Design, 139, 473-485, 2018 (lead author: M.S. student Jingran Li), Additive Manufacturing, 27, 61-71, 2019 (lead author: Ph.D. student David Garcia), Journal of Materials Research, 33, No. 1, 2018 (lead author: Ph.D. student David Garcia), and Journal of Materials Processing Technology, Vol. 303, 117528 (2022) (lead author: M.S. student Jee Yun Kim).
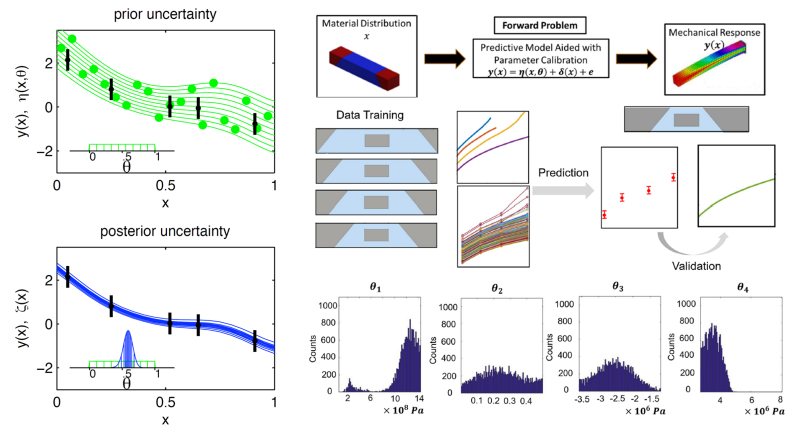
RECENT PROGRESS
Non-Planar Interface Formed by Additive Friction Stir Deposition
Mackenzie E.J. Perry
Additive friction stir deposition (AFSD) is a solid-state additive process that combines friction stir welding principles with a material feeding process to achieve site specific material deposition. The resultant strong interface bonding, no detectable porosity, and isotropic, fine grained microstructures are thought to be linked to the material flow phenomena near the interface, but experimental corroboration has remained absent. Here, we present the first comprehensive experimental study on the interface formed in AFSD, using a dissimilar Al alloy system of AA 2024 deposited onto AA 6061 substrate. X-ray computed tomography and electron backscatter diffraction show the 3-D shape of the deposited material and the concurrent microstructure evolution.
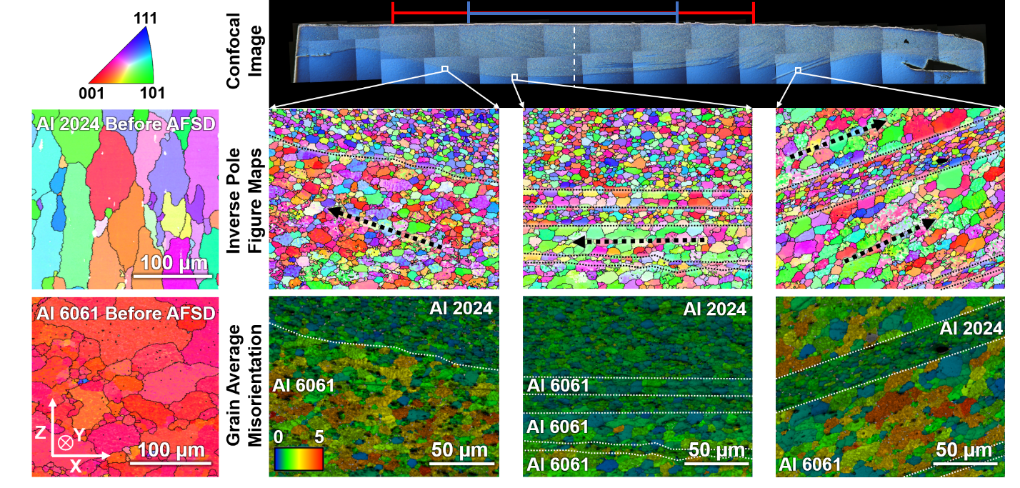
Al 2024 onto Al 6061. Video scrolls through the Z-axis, then the X-axis, then the Y-axis. Then the substrate material is removed and the deposited material is rotated 180.
Reference: "Morphological and microstructural investigation of the non-planar interface formed in solid-state metal additive manufacturing by additive friction stir deposition", Mackenzie E.J. Perry, R. Joey Griffiths, David Garcia, Jennifer M. Sietins, Yunhui Zhu, Hang Z. Yu, Additive Manufacturing, 35, (2020). https://www.sciencedirect.com/science/article/pii/S2214860420306655?via%3Dihub
Monitoring of Temperature Evolution and Material Flow during Additive Friction Stir Deposition
David Garcia
For any developing technology, it is critical to establish a relationship between the thermal process fundamentals and the equipment process variables. An extensive understanding of this relationship has not yet been established for Additive Friction Stir Deposition (AFSD) but remains necessary as the temperature evolution controls the print quality, the microstructure, and the properties of the as-printed material. Furthermore, since AFSD is a friction-stir derived technology, the heat flow and material flow are fully coupled so the distinct consideration of material flow is necessary. Here, we characterize the thermal process fundamentals of AFSD by in situ monitoring of the thermal field and material flow behavior using complementary infrared imaging, thermocouple measurement, and optical imaging.
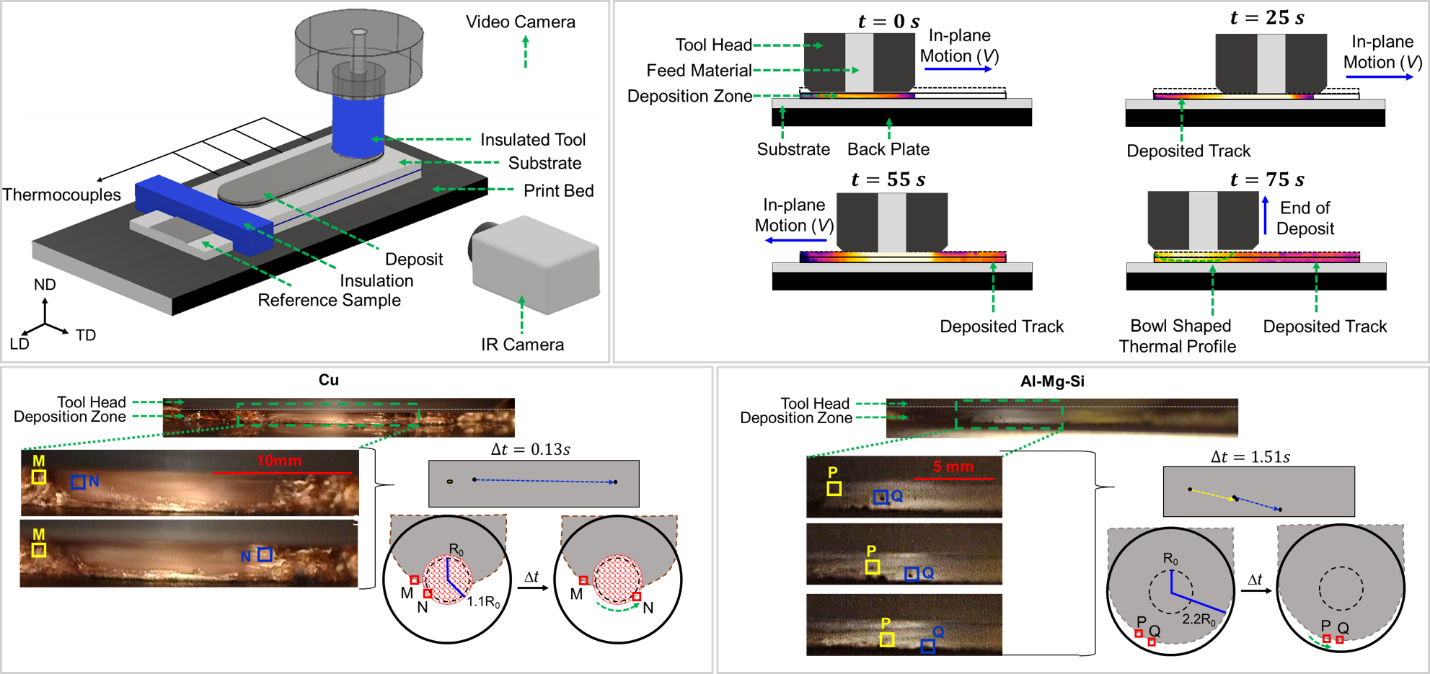
- Temperature monitoring of Cu and Al-Mg-Si reveals an increase in the peak temperature with an increase of the tool rotation rate or a decrease of the tool in-plane velocity . However, each material exhibits a distinct power law relationship: varies with in Cu but with in Al-Mg-Si.
- Optical video reveals distinct material flow behavior for Cu and Al-Mg-Si. For Cu, material rotation primarily occurs in the material directly beneath the rotating feed-rod. In AFSD of Al-Mg-Si, the tool rotation causes the entire deposition zone to rotate.
- The difference in the observed power law relationship may be attributed to differences in the heat generation mechanisms between Cu and Al-Mg-Si. As observed in the optical video, a full slipping condition occurs at the tool-deposit interface for Cu, and interfacial friction is the dominant heat generation mechanism in the deposition zone. For Al-Mg-Si, there is a partial slipping/sticking
Reference: “In Situ Investigation into Temperature Evolution and Heat Generation during Additive Friction Stir Deposition: A Comparative Study of Cu and Al-Mg-Si” David Garcia, W. Douglas Hartley, Hunter A. Rauch, R. Joey Griffiths, Rongxuan Wang, Zhenyu J. Kong, Yunhui Zhu, Hang Z. Yu, Additive Manufacturing, 34, 101386, (2020)